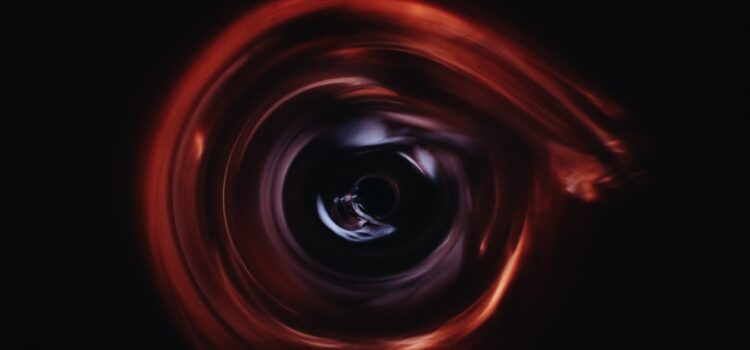
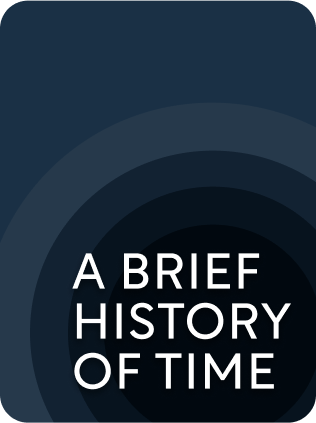
This article is an excerpt from the Shortform book guide to "A Brief History of Time" by Stephen Hawking. Shortform has the world's best summaries and analyses of books you should be reading.
Like this article? Sign up for a free trial here .
What is a black hole? How do black holes get formed? When was the first black hole discovered?
A black hole is a point in space where the gravitational pull is so strong that nothing can escape it—even light—once it enters. Black holes are formed when massive stars exhaust their supply of hydrogen and nuclear fuel and begin to collapse in on themselves under gravity.
Learn the basics of blackholes, explained in simple terms.
How Do Stars Become Black Holes?
In 1783, the English scientist John Michell wrote a paper arguing that if a star were of sufficient mass, its gravity field would be so strong that not even light could escape from it. These massive stars would be invisible, detectable only through their gravitational effects on other objects. This was the earliest sketch of what we now know of as a black hole.
How do stars become black holes? Let’s trace the life cycle of a star. Stars are first formed when free-floating hydrogen atoms begin to cluster together due to gravitational attraction. Eventually, the heat and energy from these interactions causes nuclear reactions within those atoms. Those reactions then form other elements, mostly helium. Eventually, these atoms cluster together through the force of gravitational attraction to form stars. The “outward” energy produced by the nuclear reactions that are occurring at the core of the star balances out the “inward” gravitational pressure and the star reaches a state of stability.
Eventually, however, the star exhausts its supply of hydrogen and nuclear fuel. The star begins to cool, causing it to contract. At this point, the attractive force of gravity becomes dominant and the star begins to collapse in on itself.
The Chandrasekhar Limit
Scientists once thought that, per the exclusion principle (which prevents similar particles from having the same position and velocity), the different velocities of the particles would cause the star to continue expanding, counterbalancing the attractive force of gravity. In other words, that star collapse due to gravity was impossible.
But Subrahmanyan Chandrasekhar proved that this was not the case. Chandrasekhar was an Indian graduate student who was working in the 1920s at Cambridge University. He discovered that the exclusion principle would not apply to stars that had reached a certain critical mass. He estimated that stars more than one and a half times the mass of our sun would become too dense after they had exhausted their nuclear fuel to be able to withstand the force of their own gravity. This threshold is now known as the Chandrasekhar limit.
Those stars below this limit would shrink into what are known as white dwarfs or neutron stars. In these cases, the exclusion principle would act on either the electrons or the protons and neutrons in the star to prevent total collapse, and the once-massive star would condense to a tiny fraction of its former mass—with a radius of perhaps a few thousand miles for a white dwarf and a mere ten miles for a neutron star.
But what about stars with masses above the limit? Chandrasekhar argued that such stars would collapse into a singularity of infinite density—a black hole. This prediction met with noted hostility by many prominent figures in the scientific community at the time, who refused to accept the existence of black holes.
The Point of No Return
The American theoretical physicist Robert Oppenheimer, who expanded on the theory of black holes, explained that contracting stars would impact the light cones emanating from them. A light cone, is the path of light through space-time from an event occurring at a single point in space and a single moment in time.
Oppenheimer theorized that the gravitational pull from a condensing star would bend light cones at the surface inward, making them appear dimmer and redder (because of red-shift “stretching” the wavelengths) to observers in the future light cone of the event.
At a certain point in a massive star’s collapse, the gravity makes it impossible for light to escape at all. And if light can’t escape, nothing can, because nothing can travel faster than the speed of light. Such a gravitational singularity is a black hole. The boundary of a black hole, the point past which light just fails to escape, is called the event horizon.
Hawking and Penrose’s work suggested that black holes must be infinitely dense and infinitely curved in space-time. Under such conditions, all scientific predictability would break down. “Space” and “time” would have no meaning, just like the conditions before the Big Bang. Penrose argued that singularities can’t be seen, because nothing can be observed behind the event horizon (where light cannot escape). Objects can enter the singularity but nothing can exit.
Seeing the Unseeable
So can we actually observe black holes? Because nothing can escape from them to reach us, it would seem that we could only observe their effects. In 1967, scientists at Cambridge first detected radio waves coming from a distant neutron star. This was the first absolute evidence that neutron stars were real, instead of just being predicted mathematically.
Neutron stars are objects of extremely high density, almost as dense as black holes. Remember, the exclusion principle only just prevents them from becoming a black hole singularity. Once their existence was proven, the notion of black holes suddenly wasn’t so far-fetched.
Black holes can’t be seen, but they still exert gravitational influence over other objects. This brings us to the strange case of the Cygnus X-1 system. This system consists of a star orbiting around an unseen object. Based on the star’s orbit, we know that the unseen object must have great density. The mass of the star is six times greater than the sun— too large for the unseen object it is orbiting to be anything other than a black hole.
Other likely black holes have been identified throughout the observable universe, including one possibly at the center of our own galaxy. Because of the age of the universe, there are probably more black holes than observable stars, as many stars must have undergone collapse since the Big Bang.
Low-mass stars could also form black holes, though not through internal gravitational collapse. Instead, massive external pressures could cause their compression. Such high-energy conditions would only have been possible in the early universe, the period immediately following the Big Bang. Identifying such primordial black holes could provide a window into the early universe.
Primordial Black Holes
But star collapse is not the only way black holes form. There might also be primordial black holes, formed from the collapse of irregularities in space-time just after the Big Bang, in the earliest stages of the universe. Mathematical models predict that these would be emitting gamma rays of a strength and frequency that might make them detectable.
Based on our observation of the universe’s gamma ray background radiation (which has some similar properties to the microwave background we explored earlier), we estimate that primordial black holes are rare occurrences in the universe—there would be no more than 300 of them per cubic light-year, which would mean they account for a mere one millionth of the total matter in the universe. Gamma ray outbursts have been detected, but there are too many confounding variables (such as colliding neutron stars) to confirm these bursts as coming from primordial black holes.Despite the gaps in knowledge, the proven emission of radiation from black holes has meaningful and profound scientific and even metaphysical implications. Eventually, the mass of objects that fall into the black hole is emitted as radiation. Thus, this matter is recycled as energy, until the final disappearance of the singularity. Matter is never fully destroyed, but emitted as energy. In this sense, we, being made of matter, are eternal beings.
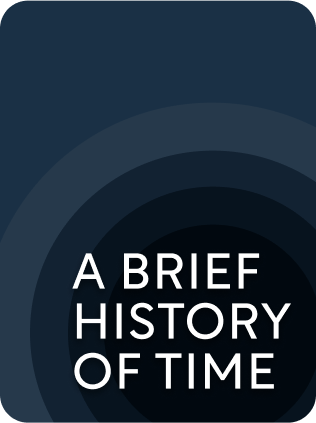
———End of Preview———
Like what you just read? Read the rest of the world's best book summary and analysis of Stephen Hawking's "A Brief History of Time" at Shortform .
Here's what you'll find in our full A Brief History of Time summary :
- The search for a theory that explains the history and evolution of our universe
- Stephen Hawking's discussions about time, space, dimensions, and quantum theory
- How time travel would theoretically work