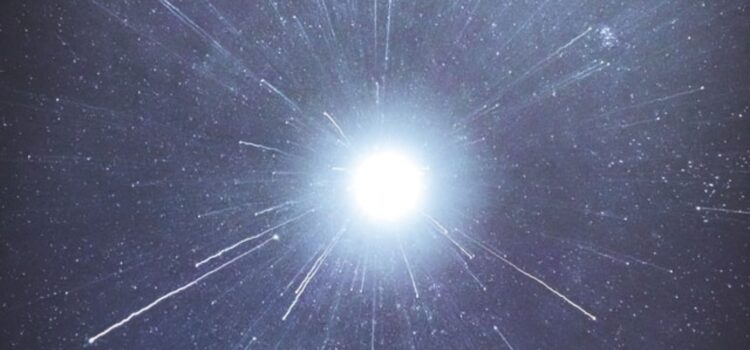
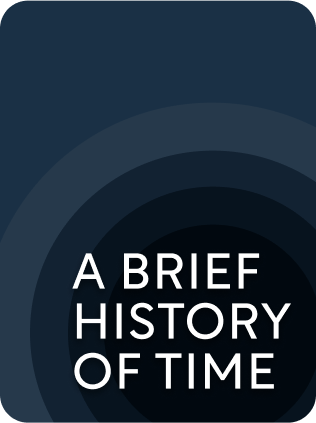
This article is an excerpt from the Shortform book guide to "A Brief History of Time" by Stephen Hawking. Shortform has the world's best summaries and analyses of books you should be reading.
Like this article? Sign up for a free trial here .
Is Stephen Hawking’s A Brief History of Time worth reading? What are some of the key topics explained in the book?
A Brief History of Time is an exploration of the forces that govern our universe. It distills a wide range of topics, from Newton’s laws of gravity to special and general relativity, the Big Bang, black holes, the possibilities of time travel, the quantum theory, and more.
Here is a brief overview of Stephen Hawking’s book A Brief History of Time: From the Big Bang to Black Holes.
Book Overview: A Brief History of Time by Stephen Hawking
Although the theoretical physicist Stephen Hawking, author of A Brief History of Time, was confined to a wheelchair and deprived of the powers of speech by the motor neuron disease ALS, he was able to advance our understanding of the universe, the nature of time, and our role within existence itself more than almost any other person who ever lived.
This overview of Stephen Hawking’s A Brief History of Time will discuss some of the key themes and ideas from the book at a high level, exploring just the main concepts and theoretical frameworks for each.
The Nature of Theories
We try to make sense of our universe by testing different theories about why we observe the phenomena we observe. The ultimate goal of all scientific endeavors is to produce a unified theory that completely explains the universe—a detailed roadmap of existence. A good theory accurately describes events, with few exceptions or changes; predicts future observations; and has the ability to be disproven in the future. Even one observation that contradicts the theory can negate it. In this sense, we never “prove” theories—we only grow more confident in them with every accurate prediction they make.
One theory of the universe that once had wide acceptance was the geocentric model of the universe, in which celestial bodies revolve around the Earth in fixed circular orbits. But this theory failed to predict the movement of the Moon accurately. Thus, it was discarded in favor of a heliocentric model, in which the Earth and the planets revolve around the sun—which lined up far better with observed reality. Subsequent theories and discoveries have enhanced our understanding.
Everything Is in Motion
Other theories that have enhanced our understanding of the universe include Newton’s Laws of Gravity. Newton’s Laws of Gravity state that force doesn’t set things in motion—everything is already in motion. There is no absolute standard of rest, because objects are always moving in relation to one another. The concepts of “rest” and “motion” are entirely relative. A stationary train may appear to be at rest, but it’s not—because it is sitting upon a moving Earth.
This relationship between the two objects (the train and the Earth) would be the same if the Earth was at rest and the train was moving. Because there’s no absolute standard of motion or rest, there’s also no absolute standard of space. It’s impossible to determine whether two events occurring at different times occurred in the same space, because everything is moving relative to everything else.
The Relativity of Time
It’s not just space—time is also relative. We know that light moves at a constant and finite speed to all observers, regardless of their position in space. But this could only be reconciled with Newton’s laws if time, too, was relative. This was the basis of Albert Einstein’s special theory of relativity, which argued that the laws of science should apply universally, regardless of the differing speed of the observers. Thus, if the speed of light is constant for everyone looking at it, then time must also be relative. This was expressed in Einstein’s famous equation e=mc2, with “e” standing for energy, “m” standing for mass, and “c” standing for the speed of light.
Thus, time is not a dimension separate from space: it is inherently interwoven with it, in the fabric of space-time. Einstein’s general theory of relativity, proposed in 1916, incorporated gravity into this framework. Under this theory, gravity is a special force that exists because of the curvature of space-time itself.
Space-time, according to this theory, is not flat. It is analogous to placing an object on a stretched-out piece of fabric. The weight of this object will cause the fabric to sink—this is the same mechanism by which gravity warps the curvature of space-time.
Universal Gravitation
In addition to his laws of motion, Newton’s laws describing gravity have also changed the way we understand the universe. Newton’s theory of universal gravitation states that bodies are attracted to one another by a force (gravity) that grows stronger in proportion to their mass and inversely proportional to their distance. But gravitational attraction, left unchecked, would eventually cause all the matter to collapse in on itself—the only way this wouldn’t happen would be if the universe was dynamic and expanding, a notion that was totally at odds with religious and theological concepts of the time.
The Big Bang
In 1823, the German astronomer Heinrich Wilhelm Olbers observed that if the universe were truly infinite, static, and eternal, the sky would be blinding white light—because in an infinite universe, there would be an infinite number of stars. Every line of sight would end at a star. This paradox pointed the way toward an understanding of the universe as finite, expanding, and with a definite beginning point in time. The reason we aren’t blinded by light when we gaze at the sky is because the light from some stars hasn’t reached Earth yet. And if this is the case, then the universe must be finite and there must have been some point at which the stars “turned on” and the universe began. This beginning is what we now know as the Big Bang.
In 1929, the American astronomer Edwin Hubble confirmed through observation what Olbers’ paradox had suggested. Hubble discovered that the universe was expanding by observing the “red-shifting” of distant galaxies—the movement of their light toward longer, red wavelengths, indicating movement away from us.
The Beginning of Time
The theory that the universe is expanding explained what happened after the Big Bang. But what happened before?
Events “before” the Big Bang are inconsequential because they are, by definition, unobservable and unable to affect anything that came “after” the Big Bang. Indeed, the very concept of time itself can be said to have come into existence at the Big Bang.
But did general relativity and the existence of an expanding universe require a Big Bang event? The British mathematician and physicist Roger Penrose sought to answer this in 1965. Reasoning from general relativity and the principle that gravity is always attractive, Penrose theorized that when a star died and collapsed under the weight of its own massive gravity, it would be compressed to a space of zero surface and volume—a singularity, whose conditions would be much like those before the Big Bang. The great insight of Stephen Hawking, a colleague of Penrose’s, was to put Penrose’s theorem in reverse—If all stars ended up as singularities, then an expanding universe must have begun with a singularity.
Star Death
Stars are massive bodies with enormous gravitational pull. What keeps them from collapsing in on themselves during the majority of their lifetimes are the nuclear reactions that are occurring at their cores—this “outward” pressure balances the “inward” gravitational pressure.
Eventually, however, the star exhausts its supply of hydrogen and nuclear fuel. At this point, the star begins to cool, causing it to contract. Stars that are more than one and a half times the mass of our sun collapse into a singularity of infinite density—a black hole. A black hole has infinite gravity, such that not even light can escape. Under such conditions, all scientific predictability breaks down. “Space” and “time” have no meaning, just like the conditions before the Big Bang.
Black holes can’t be seen, but we know they exist, because they exert gravitational influence over other objects. Scientists have detected them in the distant Cygnus X-1 system and possibly at the center of our own galaxy. Because of the age of the universe, there are probably more black holes than observable stars, as many stars must have undergone collapse since the Big Bang. Black holes could also have been created under the high energy and density conditions of the early universe.
The State of the Universe
Although there’s evidence the universe is currently expanding, does general relativity actually predict an eventual gravitational collapse of the entire universe, also known as a “Big Crunch” event? Are our universe’s days numbered? For most of the 20th century, the “Hot Big Bang” model, a variant of the Big Bang theory, has been used to tell the story of the origin and development of the universe and answer these questions.
According to the Hot Big Bang model, after the initial Big Bang explosion, the universe was incredibly hot (hence the name), meaning that particles were moving too quickly to merge together to form protons, neutrons, atoms, and molecules. But as the universe expanded, it began to cool, and the particles slowed down. This led to a series of nuclear fusions, in which stars formed, as well as more complex elements like hydrogen and helium (this all would have taken place within mere milliseconds after the Big Bang). After just a few hours, most of the hydrogen and helium in our universe today was created, concentrated in enormous clouds. These then became the building blocks of the other elements and molecules that form all the matter in our universe (including Earth and human beings).
But it all seems too perfect. The universe seems too uniform (at least on a large scale) to be the product of an explosion like the Big Bang. Moreover, it’s curious that the rate of expansion is just large enough to avoid gravitational collapse. After all, if the initial rate of expansion after the Big Bang had been only infinitesimally smaller, the universe would have already collapsed.
Alan Guth at MIT suggested an “inflationary” hypothesis to explain this puzzling state of the universe, without reverence to a deity or intelligent being carefully setting such conditions.
This theory tells us that the expansion after the Big Bang was so rapid that the universe ballooned to a million million million million million times its radius in less than a second. This expansion would have supercooled the universe much faster than in the traditional “Hot” Big Bang model and produced a great smoothing and uniformity across the universe on a large scale, even if it didn’t start out uniform and smooth.
Entropy and Backwards Time Travel
We’ve already established that time is relative. But this just raises another major question about our universe—if time is relative, in what direction does it point? Can it possibly move backwards?
Unfortunately for those who might wish to journey to the past, there are three reasons to suggest that this is an unlikely possibility.
Reason #1: The second law of thermodynamics says that, within a closed system, total entropy, or disorder, can only increase or remain stable over time—it can never decrease. But the backwards movement of time would represent an inversion of this law. Under such conditions, we would witness the decreasing of entropy over time—a broken cup, for example, becoming an intact cup. We don’t observe the spontaneous reconstitution of broken cups into whole ones, thus the thermodynamic arrow of time moves only in a forward direction.
Reason #2: Time also moves only in a forward direction in a psychological sense—we don’t remember events in the future and always perceive ourselves as moving toward the unknown future and away from the known past. This also happens to be the direction in which entropy increases. This is the psychological arrow of time.
Reason #3: Time moves only in a forward direction because entropy only increases in the same direction as the expansion of the universe. We can only exist in the expanding phase of the universe, when entropy is increasing. This is the cosmological arrow of time.
Paradoxes of Backwards Time Travel
To travel back in time, one would need to be able to travel faster than the speed of light. Imagine you were in a rocket travelling from point A to point B. Under the laws of general relativity, all observers would be able to agree that your rocket was at point A before it reached point B—provided that it traveled below the speed of light. But if it were travelling faster than the speed of light, your rocket would outpace its own light being transmitted to observers at point A. Once you arrived at Point B, you would be able to observe your past self leaving Point A. You would be at your destination before you left—in other words, you would have traveled backwards in time.
But physics seems to disallow this, because, according to Einstein’s famous equation e=mc2, mass increases with speed, and you would need more and more energy to power a rocket past the speed of light, and there will never be enough energy to accelerate beyond this point. Lastly, there are the inherent paradoxes baked into the idea of backwards time travel. Perhaps the most famous is the “grandfather paradox,” in which you go back in time and kill your grandfather, which would mean you didn’t exist to travel back in time, which would mean your grandfather is still alive, and so on. The only way around this would be a scenario in which backwards time travel does not allow one to alter the course of events because one enters a zone of alternate history that cannot impact our space-time.
Matter and Antimatter
We’ve explored general relativity and its implications for the origins and state of the universe. But there is another, incompatible theory of the universe—quantum theory. According to quantum theory, waves release energy in discrete quantities (called “quanta”) that are directly proportional to their frequencies. In order to measure the position and speed of any particle, one would need to shine a quantum of light on it—but doing so alters the position and speed of the particle one is attempting to measure. This is the foundation of Werner Heisenberg’s uncertainty principle, which states that it is impossible to accurately gauge both the position and speed of any given particle.
This is the cornerstone of the field of quantum mechanics, which predicts a range of possible outcomes for all phenomena in the universe (since the universe is, ultimately, composed of particles), and tries to work out probabilities, rather than searching for single, definitive predictions. This insight shaped so much of what we have come to understand about particles, the building blocks of our universe. By the early 20th century, scientists had worked out the basic atomic structure: a positively charged nucleus (consisting of a positive proton and a neutral neutron) orbited by a number of negatively charged electrons. In the 1960s, scientists discovered quarks, the particles that make up protons and neutrons.
All types of particles have a corresponding antiparticle. Antiparticles make up what is called antimatter. For instance, the electron has the positron, and the two can annihilate each other. Interactions between matter particles (mainly protons and neutrons) happen via force particles (those particles that make up forces like gravity and electromagnetism). Although these force particles can’t be detected, we know they exist because of their measurable effects on matter particles.
So if there is such a thing as antimatter, how much of the universe is made of it? Can we detect it? We believe that the universe is made overwhelmingly of matter—quarks and the protons and neutrons they form, as opposed to antiquarks, antiprotons, and antineutrons. Otherwise, we would be seeing radiation from the annihilations that would be happening from matter colliding with antimatter. The very existence of observable matter proves the relative scarcity of antimatter in the universe.
Beyond Four Dimensions
String theory replaces the concept of particles with strings—instead of a particle occupying a single point in space at a single point in time, there are strings occupying a line in space across each point in time. Thus, all points correspond to a time and to a position on the string.
String theories shatter the longstanding notion of four-dimensional space-time. For them to work at all, string theories require up to 26 dimensions of space-time. We don’t see the extra dimensions because they are infinitesimally small. They are tiny warps or curves in space-time, far too small to be observable on a large scale.
So why don’t we see these extra dimensions or the regions of space-time with fewer than three? Because two spatial dimensions would be insufficient to support life (the world would look like a side-scrolling video game, and blood circulation would be impossible). So would any more than three spatial dimensions, because they would destabilize the revolution of the Earth around the Sun. Thus, we observe our four flattened dimensions because those are the only regions of the universe where we can possibly exist.
The Final Picture
We know that the uncertainty principle hems in our predictions of particle behavior (and thus, the universe). Thus, we have modified our aims and now try to describe the universe and predict events only within the bounds prescribed by it.
We might never know if we discover the unified theory, because theories, by definition, can’t be “proven.” But if our predictions consistently lined up with observations, we would have good confidence that we had found such a theory. Even though such a theory would, at first, only be known to a handful of elite specialists, this knowledge would eventually be disseminated across the human population. General relativity and quantum theory, too, were once the provenance of only the most cutting-edge theoretical physicists; today, they are taught to millions of college students around the world. And it would mean that humans would possess a complete understanding of the events around us and the laws that govern our universe. It would be the fulfillment of man’s eternal quest for knowledge.
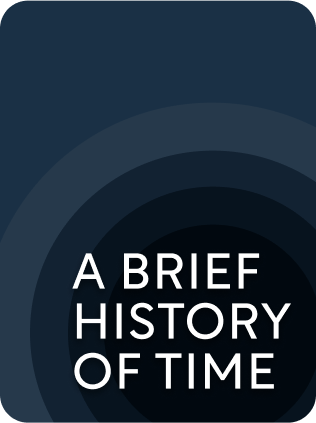
———End of Preview———
Like what you just read? Read the rest of the world's best book summary and analysis of Stephen Hawking's "A Brief History of Time" at Shortform .
Here's what you'll find in our full A Brief History of Time summary :
- The search for a theory that explains the history and evolution of our universe
- Stephen Hawking's discussions about time, space, dimensions, and quantum theory
- How time travel would theoretically work