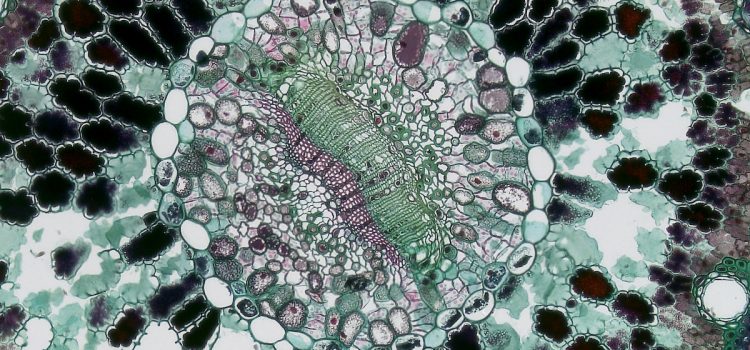
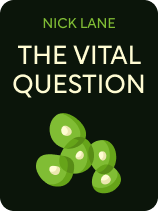
This article is an excerpt from the Shortform book guide to "The Vital Question" by Nick Lane. Shortform has the world's best summaries and analyses of books you should be reading.
Like this article? Sign up for a free trial here.
How is a cell made? What does this knowledge tell us about life’s origins?
To deduce how life began, biologists like Nick Lane have to reverse-engineer our primordial single-celled ancestors. Working backward through time, it’s possible to hypothesize the traits of the very first microbial life.
Keep reading to understand what we know about how cells are made.
How Is a Cell Made?
Dubbed the last universal common ancestor, the first cell contained only the biological features found in all living cells today but not characteristics that evolved later—such as different species’ internal chemistry or the different ways that cells divide.
How is a cell made? Lane discusses the minimum structural requirements a living cell needs to survive and reproduce, the environmental factors that must have been present for life to arise from nonliving matter, and the sequence of events that may have taken place to ignite the first spark of life.
What a Cell Needs
The most easily identifiable parts of a cell are the self-replicating molecules that encode its chemical blueprint—DNA and RNA. The next requirement that makes a cell a “cell” is a physical structure to define its boundaries. Beyond that, a cell needs a steady supply of food and energy, as well as some kind of catalyst for metabolic reactions (which break the food apart into building blocks and ATP). Finally, the cell needs a way to eject the waste products of its metabolism so that they don’t clog the cell with useless matter. Lane says all these features had to be there from the start, which returns us to the question of which came first—the structure of the cell or the DNA blueprints for how to build one?
(Shortform note: Though Lane discounts the “information first” hypothesis for the origin of life, he doesn’t address the question of what information the first DNA carried if not the instructions for building a cell. The most likely answer is that prebiotic DNA was gibberish—mere random assortments of polymerized nucleotides that facilitated the production of other organic compounds around them. Some of those organic molecules became the enzymes that unravel DNA and build each separate strand into a new DNA molecule.)
Lane suggests that the answer to the riddle is that life arose in an environment where many of its necessary components already existed in a nonliving form. We know that matter can spontaneously organize itself, given enough energy input. For example, take giant rogue waves—unpredictable, ship-wrecking swells that spontaneously emerge when smaller waves and ocean currents collide deep at sea, suddenly self-organizing into mountains of water as their molecules’ energies add to one another. In the right circumstances, this kind of interaction can take place on the microscopic level, where flows of hot, chemically saturated water give rise to organic compounds such as amino acids, fatty lipids, and even self-replicating RNA.
(Shortform note: As explained in our discussion of thermodynamics, microscopically matter self-organizes when atoms align into crystal structures. We also observe self-organization on the largest scale—galaxies that develop complex spiral structures over the billions of years of their existence. In general, systems of every scale self-organize when feedback loops of cause and effect amplify and reinforce features that were initially random patterns. For example, one driver slamming their brakes causes other drivers to hit their brakes as well, organizing a spontaneous wave of slow traffic according to the laws of fluid dynamics. The feedback loops of organic and geological chemistry are what Lane says can generate life.)
Cells Without Borders
While the same basic organic compounds are common to all known forms of life, one trait that isn’t shared between bacteria and archaea is the structure of the outer cell wall. Both have them, but both have significant differences on the molecular level—differences strong enough to suggest that the two domains’ cell wall structures developed independently from each other in a case of parallel evolution. From this, Lane concludes that the original cell didn’t have an organic cell wall at all—the cell wall evolved later in the evolutionary time frame. Since the first cell had to have some kind of boundary, that boundary must have been inorganic, perhaps even geological in nature, which gives our first clue as to where life may have formed.
Parallel and Convergent Evolution If, as Lane suggests, bacteria and archaea evolved their cell walls independently of each other, this would be an example of parallel evolution, such as unrelated American and African desert plants that developed similar adaptations to survive in arid climates. This becomes convergent evolution when adaptations over time make unrelated species resemble each other. In this case, the similarities aren’t due to a shared genetic ancestor, but to the fact that in each species, evolution is trying to solve the same basic engineering problem. The most striking example of both parallel and convergent evolution is the development of the eye across many different classes of animal life. Beginning from the biological problem of how to detect light and use it to survive, the animal kingdom has come up with at least 10 different types of designs from more than 50 different points of origination. Results include the color vision of primates, the compound eyes of insects, the eyestalks of mollusks, the pinhole eyes of clams, and the light-sensitive cells of jellyfish. |
The second clue to where life may have formed is the electrochemical membrane gradient that’s used to power all living cells. The original cell must have been born in a place where such gradients can occur naturally. Finally, there’s the requirement for the cell to metabolize food into the molecular building blocks it needs to grow and reproduce. In modern cells, the flow of food and waste into and out of the cell is guided by complex, specialized proteins that have evolved over eons. Lane argues that before life began, those specialized proteins couldn’t have evolved yet, so something else must have directed that flow—perhaps a stream of heated organic compounds funneled through a constricted passage.
(Shortform note: One component absent from the conditions Lane describes is the presence of light, which implies that life could rise in places where sunlight is hard to come by. This is why planetary scientists believe that one of the most likely candidates for non-terrestrial life in our solar system is the subsurface ocean of the icy moon Europa, whose rocky core is heated by tidal squeezing from nearby Jupiter. Since the atomic ingredients of life are common throughout the universe, the only questions are whether Europa has alkaline vents with organic molecules and whether a similar series of events took place there as did on the early Earth.)
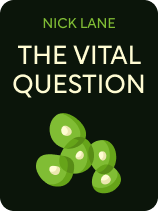
———End of Preview———
Like what you just read? Read the rest of the world's best book summary and analysis of Nick Lane's "The Vital Question" at Shortform.
Here's what you'll find in our full The Vital Question summary:
- Why genetics alone aren't enough to explain why cells function as they do
- A theory on where all complex life originated from
- An explanation of how cells work and are made